Colour and art: notes for a talk delivered at the V&A Museum, London, October 2002.
Download PDF or Word Doc.+ Powerpoint (ZIP file 6.4Mb)
COLOUR, ART AND SCIENCE – Philip Ball
Notes for a talk delivered at the Victoria & Albert Museum, London 20 October 2002
There is a Powerpoint presentation to accompany this talk
Fundamentals of colour science
What is colour?
An intrinsic property of substances (Aristotle)
Aristotle believed that light is something transmitted from an object to the eye. The colour of the object is then an intrinsic property, like its weight or taste.
Something substances do to light
Aristotle believed that the rainbow’s colours were not like normal colours: they are caused by reflection of sunlight from raindrops in distant clouds. He reasoned that each droplet acts like a tiny mirror, and that such mirrors can change white light into coloured light. This gave rise to the idea that colour arises when objects somehow alter light.
Goethe’s theory of colour builds on this idea. He regarded light as some kind of fundamental and homogeneous thing – a kind of universal radiance – that becomes coloured when disturbed and modified by differing degrees of darkness. So in Goethe’s model there are two polar extremes: light and dark, with all the colours arrayed between them. This is similar to the ancient Greek view of colour.
But rather confusingly, he somehow equated light with yellow (there was a common view that sunlight was yellow) and dark with blue, and suggested that red could be made by mixing yellow and blue. Goethe’s influence on artists, e.g. Turner, Kandinsky, theosophy & Mondrian. [Images: Turner’s Deluge (1843), Kandinsky, Mondrian]
Light itself (Newton)
Misconception that Newton in the 17th C showed that ‘white’ sunlight could be split by a prism into the visible spectrum. In fact, this was long known – even to Aristotle. But the idea was that the prism was somehow modifying the light. Newton showed that it was in fact revealing the components of white light. He showed that by using a lens to refocus the spectrum, one could reconstitute white light. And he showed that the individual colours were irreducible.
So Newton turned colour theory away from substances and towards light.
What is light?
Electromagnetic spectrum (Maxwell). Newton divided the spectrum into seven fundamental colours – arbitrarily. He recognized that the extreme ends of this spectrum faded into similar colours – red and violet – and he united them in the colour wheel. Later colour wheels were simpler and more symmetrical: six colours. The modern colour version of the colour wheel is the CIE diagram (Commission Internationale de l’Eclairage), which is less elegant but more scientifically informative.
Additive and subtractive mixing
To many artists, Newton’s theory of colour was completely unsatisfactory. In particular, it said strange things about colour mixing: that all colours mix to white. And light mixes in other strange ways: red and green make yellow, for instance. This is one of the principal objections of Goethe to the Newtonian theory.
Painters knew that almost any colour could be made by mixing just a few ‘primaries’. Since the 17th C, these were generally regarded as red, yellow and blue, with white and black for lightening and darkening. But in the mid-19th C, Maxwell showed that he could make light of any colour by mixing three different primaries: red-orange, blue-violet and green. Mixing light is not like mixing pigments (additive and subtractive).
Complementary colours
Artists and designers had known for a long time that certain colours go well together – they seem to enhance one another. In the 19th C, the French chemist Michel-Eugene Chevreul formalized these relationships in his theory of ‘simultaneous contrasts’. He was the director of the Gobelins dye works in Paris, where he was set the task of finding out why the dyes seemed dull. He realised that there was nothing wrong with the dyes themselves, but that threads of complementary colours were being woven too close together. When that happens, our eye can’t resolve them and they merge into a kind of greyness. But if the coloured patches are bigger, the colours look brighter. And at the point just before they start to merge, they seem to shimmer. This effect was sought after by the Impressionists, who often placed patches of complementary colours together to enhance their brilliance. The shimmering effect was what George Seurat and the other Neo-Impressionists were looking for in their pointillism technique.
Colour and perception
One thing we do have to thank Goethe for is the notion that colour is as much about perception as about light. It’s not just about what reaches our eye, but how we perceive it. Red and green light remain just that when they are mixed: they don’t turn into light of a ‘yellow’ wavelength. It’s our visual system that turns the effect of red and green light into a sensation of yellow. Maxwell agreed with this: he said ‘The science of colour must· be regarded as essentially a mental science.’
Just a brief word about how colour vision works. The English scientist Thomas Young suggested at the start of the 19th century that, rather than having cells sensitive to every different wavelength of visible light, we had just three types, sensitive to the three primary colours, and the sensation of colour came from the mixture of responses from each cell type. The German physicist and physiologist Hermann von Helmholtz later showed that this was essentially correct. We have three types of colour-sensitive cells in our retinas (cones). It’s usually said that these are sensitive to red, green and blue light; actually, their strongest sensitivities are to violet, yellow and green. And there’s nothing fundamental about the fact that there are three types – colour vision is possible with only two types of cell, and in fact most New World monkeys have only two. Some animals – some fish and birds – have four types of cone cell, making their colour discrimination more acute than ours
Causes of colour
e.g.:
Adsorption (pigments, dyes)
Diffraction (butterflies, iridescence)
Refraction (rainbow)
There are many different ways in which colour can be generated. In looking at colour in art and design, we are usually concerned with how different substances absorb light. When white light falls on an object, it might absorb some wavelengths and reflect others. We see the reflected light, and that’s what determines the colour we perceive. A green object absorbs red and blue light – chlorophyll, the main pigment in plant leaves, does this. A red object absorbs much of the green and blue, and so on. What colours a substance absorbs depends on its chemical components. Certain metal atoms tend to absorb certain wavelength bands, creating distinctive colours. Cobalt, for instance, absorbs the longer-wavelength light and reflects blue. Copper atoms typically reflect greenish-blue light (think of verdigris); iron compounds often reflect reddish light (think of blood and rust).
But colour can also arise from the way light rays, with their regular undulations, interact with grids or other regular patterns, when the pattern repeats on the same kind of scale as the wavelength. Here’s an image of the scales on butterfly wings under a microscope: you see these grooves. These reflect some wavelengths more strongly and others, creating colours. Blues and greens on insects are often produced this way. If you look from different angles, the colour changes slightly, causing iridescence.
And a rainbow’s colours are produced by another light phenomenon, called refraction: basically the same as what happens in a prism, where light of different wavelengths is bent through different angles.
Colour technologies
Most technological methods for making colours, at least until the 20th C, have relied on either using naturally coloured substances such as minerals and dyes, or finding chemical ways to make synthetic coloured materials.
I want to stress that even using natural colours was generally a technological process. Traditionally, many pigments have been ground-up minerals such as ochres (iron oxides), chalk, malachite and azurite. But getting at these minerals was no easy matter. Pic from Agricola – shows what a technological process mining was. In fact, mining was the driving force for several technical innovations in engineering.
But even from the earliest times, artists have used whatever capabilities their technology makes available to produce new colours. There is evidence that Stone Age artisans used fire to heat up a natural iron mineral called goethite, to convert it to another mineral called haematite, which produces a finer shade of red. In ancient Egypt there was already a well-developed chemical technology for making colours. One of the most renowned was Egyptian blue, which is a kind of ground blue glass made from limestone, quartz (sand) and a copper mineral such as malachite. The copper gives it its colour. The Egyptians began by making a blue glaze from copper minerals, soda and sand, which they coated on carved stone ornaments called faience. Experiments in faience manufacture probably led not only to the invention of Egyptian blue but to the discovery of glass itself, and perhaps even to the discovery of copper smelting from its ore – the innovation that produced the Bronze Age.
The key point here is that experiments and advances in colour making were driven by a social demand for more mundane substances such as glass and glazed ceramics. Most of the early technical advances in chemistry, without which colour manufacture would not have been possible, came from industrial processes like this, including soap-making and textile dyeing.
There are several examples of this in the British Galleries, e.g. the sixteenth-century ceramic mug glazed a deep blue, made in the Netherlands. The label calls this a ‘lead-glazed mug’, but in fact the blue colour of the glaze comes from the addition of cobalt. Cobalt is a metal, but it was barely recognized at that time as an element in its own right: German miners regularly dug up cobalt ore, but they simply regarded it as a kind of mineral, without thinking too deeply about what was in it. Nevertheless, cobalt minerals were widely used to make these blue glazes. They had also been used since ancient times to make blue stained glass. And since at least the 14th C this blue glass was ground up by European painters to make a relatively cheap blue pigment called smalt.
In the late 18th C, a French chemist called Louis-Jacques Thénard was asked by the French government to try to find a substitute for the very expensive fine blue pigment ultramarine. He knew that the potters of Sevres used cobalt minerals in their glazes, and so he investigated the chemistry of cobalt compounds and came up with a rich blue crystalline material called cobalt aluminate, which became known as the pigment cobalt blue. Here we see glass-making, ceramics and painting all feeding off one another.
The finest blue pigment even in the 19th century was ultramarine. This is a ground-up form of the mineral lapis lazuli, which since the Middle Ages was imported almost entirely from mines at Badakshan in Afghanistan. That is partly what made it so expensive; but it was also very laborious to make. [Explain how] So painters in the Middle Ages and the Renaiisance had to be very adept at handling their materials.
It wasn’t until the 19th C that chemists worked out how to make ultramarine synthetically, so that they no longer had to rely on rare natural sources. Again, the crucial clues about the chemical composition of ultramarine came from analyses of blue materials found in the kilns of glass factories. The French Society for the Encouragement of National Industries offered a big financial reward for the first person who could come up with a method for manufacturing this pigment industrially, and the reward was claimed by a French chemist in 1828. Thereafter, artificial ultramarine was commonly called French ultramarine. Some painters, including Turner, never really trusted it, although it was considerably cheaper and really just as good as the natural mineral. It’s the blue that Yves Klein used for his famous monochromes.
Pigments in the 19th C
As far as pigments were concerned, the 19th C changed everything. That was when chemistry became a modern science: when scientists began to understand what the chemical elements are and how they combine. Several new elements discovered in the late 18th and early 19th centuries turned out to produce brightly coloured compounds that could be used as pigments – chief amongst them cadmium and chromium.
Dates of pigment innovations:
1704: Prussian blue (iron hexacyanoferrate)
1775: Scheele’s green (copper arsenite)
1802: cobalt blue (cobalt aluminate)
1804: chrome yellow and chrome orange (lead chromate)
1814: emerald green (copper aceto-arsenite)
1819: cadmium yellow and cadmium orange (cadmium sulphide)
1828: artificial ultramarine 1834: zinc white (Chinese white) (zinc oxide)
1838: viridian (hydrated chromium oxide)
1859: cobalt violet (cobalt phosphate/arsenate)
1860s: cerulean blue (cobalt stannate) 1861: aureolin (potassium cobaltinitrite)
Note that these include the first strong, pure and stable orange and violet pigments available to artists.
These changes transformed the artist’s palette. Those who made use of the new, brighter pigments were often stylistic innovators too: first Turner, then the Pre-Raphaelites, then the Impressionists. Typical Impressionist palette: 12 out of 20 of the colours are inventions of the 19th C.
Specialist paint manufacturers began to appear in the 18th C, evolving out of grocers who also sold spices and drugs. By then painting had become a fashionable pursuit of the well-to-do, and numerous manuals were being written that were aimed at this amateur market. This niche was also exploited by the artists’ suppliers. Paints were manufactured using mechanized grinding equipment powered by horses and later by steam. For fine art they were mixed with oils, but for the amateur market a more portable and less messy form of paint was produced: the watercolours. These are basically pigments suspended in a water-soluble gum, generally the Acacia extract gum arabic. There is an example of a watercolour set in the British Galleries, made by Reeves and Woodyer around 1820. This company is one of the oldest specialist paint manufacturers, established by Thomas Reeves in London in 1766. Here’s an example, from the Morning Herald in 1790, of the hazards they faced in 18th-C London:
“Yesterday an overdrove ox ran into the shop of Mr Reeves, Colourman to her Majesty, Holborn Bridge; broke the windows of the shop and knocked down near the whole of the colours and stock; he was at last secured and taken to the slaughter house. He tossed two women in Holborn and bruised them in so dreadful a manner that they were conveyed to an hospital without hope of recovery.”
Show also the exhibits of watercolour paints for painting velvet, made by Reeves & Woodyer in 1814 – another genteel pastime.
Note that the demand for pigments was also hugely buoyed by the interior-decorating market. House paints became popular since at least the sixteenth century. One of the most commonly used for the walls of well-to-do houses was a cheap blue called blue verditer, an artificial copper-based byproduct of silver refining, sometimes called blue bice. Verditer itself derives from ‘vert de terre’, French for ‘green earth’ – because the same industrial process could also produce a green copper pigment. Blue verditer wasn’t an especially distinguished colour – you can see what it might have looked like from the reconstruction of the Drawing Room at 11 Henrietta St in the British Galleries, dating from the early 18th C. Here the walls have been covered in a slightly greyish blue cloth which is probably close to the kind of hue verditer would produce.
Dyes
Colour in the British Galleries has a far greater emphasis on dyes than on pigments. Traditional dyes are nearly all organic compounds – that is, they are carbon-based molecules, and were extracted from living organisms.
e.g. Indigo: from a plant called Indigofera tinctoria, grown in India and imported by the Romans.
Madder: from the root of the madder plant Rubia tinctorum, which was cultivated in Europe from at least the 13th C. [See Great Bed of Ware – madder and weld] Cochineal: an extract of insects. This was first extracted from insects found on plants grown in Poland around the fifteenth century. It has a deep crimson colour, and was labour-intensive to collect and very expensive. The Spanish invaders of Central America in the early sixteenth century found that the Aztecs extracted a very similar dye from insects dwelling on cactus plants, and by the latter part of the century most cochineal came from the New World. The velvet bearing cloth from the late 17th C in the ‘Birth, marriage and death’ section of the British Galleries is probably dyed in cochineal, although this hasn’t been analysed for certain.
These crimson dyes were considered the finest and most luxurious colours for dyeing in the Middle Ages and the Renaissance. They were used for the ceremonial robes of kings and priests; the deep crimson alluded to the legendary Imperial purple of Rome. This is Raphael’s portrait of Pope Leo X (c.1517), wearing robes probably dyed with cochineal.
It’s interesting how one might translate this dye colour into a pigment colour for painters. Dyes are too translucent to be used directly in oil paints, but they could be fixed to the surface of particles of white mineral powders such as alumina (aluminium oxide) using chemical processes, to make a so-called lake pigment. These techniques weren’t perfected until the late Middle Ages, so it wasn’t until then that painters had a good deep red available. It’s interesting to compare this picture with that of Thomas More and Family, painted in 1593-4 after a version by Hans Holbein in 1527. Here Thomas’s father John wears the red robes of a judge of the King’s Bench. This would probably have been dyed with the insect-based dye called kermes. But in the painting it looks more orangey than crimson, and I suspect it might be painted using the more common red pigment vermilion. This shows how the availability of materials to artists circumscribed their ability to paint what they saw.
Textile dyeing and colouring was an immensely important industry – probably the biggest single manufacturing sector of the British Industrial Revolution – and was even more important than pigment manufacture in stimulating new research and discovery in colour chemistry. We can tend to think of traditional dyeing as a quaint folk craft, but in fact it was already a well organized industry by the late Middle Ages, with its guilds and regulations. One of the cheapest mass-produced clothing textiles in the Industrial Revolution was cotton, and there was a craze for printed calico. Dyeing is a subtle chemical process: one needs to use a mordanting agent which enables the dye to stick fast to the thread. Until the 19th C, mordants were generally found by trial and error.
Printing of patterned textiles could be done in two main ways: either by covering parts of the cloth with pastes and waxes, called resists, to protect them from the dye, which was taken up in the exposed areas, or by printing the fabric using wooden blocks coated with inks or oil paints. Block printing and dyeing with resists were often seen as separate trades, with their own guilds. Dyers often used blue dyes like indigo and woad, so this method was called blue printing. After the late 17th C, block printers commonly followed the practices used for patterning textiles in India, which typically employed madder red and produced textiles called chintz. There was a craze for imported chintz in the late 17th and early 18th C, and the Indian printing techniques were more advanced than those in Europe: they had perfected methods of multicolour patterning. [Example from c.1700 in British galleries.]
Multicolour printing, mordanting and bleaching all required some degree of chemical expertise, and so textile companies in the 18th C began to employ knowledgeable chemists. Working in the textile printing and dyeing business began to be seen as a good career option for chemists. This interaction stimulated the invention of new dyes, made not from natural extracts but from raw chemical ingredients. This was the start of the modern chemical industry.
One of the most important sources of raw materials for chemists insterested in making new organic compounds was coal tar, the residue produced by coal-gas works that proliferated thanks to the demand for gas lighting. When coal tar was distilled, all sorts of new compounds could be extracted from it, such as benzene and phenol. Phenol was used in the 1840s to make a new yellow dye for silk, called picric acid. In the 1850s there was a fashion for purple, due partly to a dye called murexide made from solidified guano imported from Peru. [Show Arthur Hughes’ April Love, 1856.]
That year was a turning point for the dye industry. One of the leading experts on coal-tar extracts was the German chemist August Wilhelm Hofmann, who was director of the Royal College of Chemistry in London. He set one of his young students, an 18-year-old called William Perkin, the task of trying to make the antimalarial drug quinine from a coal-tar extract called aniline. In 1856 Perkin tried and failed; instead he found that he’d made a rich purple compound which dyed silk very effectively. Perkin took this substance to the Scottish dyers for testing, and even though they found it was hard to get it to stick to cotton, he then persuaded his brother and father to set up business to manufacture the purple as a dye. It became known as mauveine, or later just ‘mauve’, the French word for mallow.
This was the first coal-tar dye. It caught on within two years, and soon Perkin’s mauve was everywhere in fashionable society. Around the end of 1858 a French chemist discovered a red aniline dye, called fuchsine. The next year, two former students of Hofmann’s, named Edward Chambers Nicholson and George Maule, set up a business making coal-tar dyes with a paintmaker named George Simpson. Very soon, other new dyes were discovered: magenta, aniline violet, aniline blue. And aniline dye companies began to spring up all over Europe. In Germany, Friedrich Bayer began in 1862, and the Badische Anilin und Soda Fabrik in 1865. These are now Bayer and BASF, two of the biggest chemicals manufacturers in the world. The chemicals companies Ciba-Geigy, Hoescht and Agfa also began as dye manufacturers.
There is an article on display in the British Galleries by the eminent English chemist William Odling which recounts the history of the coal tar dyes. It is in the Practical Mechanics Journal, and shows specimens of some of the dyes made by Simpson, Maule & Nicholson. And this is also from the galleries [pic], a sampler of the dyes made by Friedrich Bayer. These coal tar dyes had stronger colours than most of the traditional animal or vegetable dyes. Some of them weren’t actually very good – they washed out or faded in sunlight. But bit by bit, the synthetic dyes got more reliable, and chemists began to figure out how to tinker with the chemical composition of the molecules to make new colours almost to order.
The traditional dye industry had relied on cultivation of plants like indigo and madder on a large scale. Now that was under threat. And it got worse. In 1868 two German chemists worked out how to make the molecule that gave madder red its colour, called alizarin. This meant it was no longer necessary to grow madder – an expensive and uncertain business. Instead, it could be put together by chemical treatment of coal-tar extracts. By the 1880s, madder cultivation was on its last legs. The same thing happened to indigo when BASF developed a way to manufacture it synthetically in the late 1890s. Soon the giant dye companies began to diversify into new chemical products, such as pharmaceuticals – some of the first chemical drugs, such as the anti-syphilis agent Salvarsan and the first artificial antibiotic Prontosil Rubrum, were basically synthetic dyes. And that, I suppose, was the beginning of the profusion of colour that we see around us today.
AMAZON.co.uk – Bright Earth: Art and the Invention of Colour – Philip Ball
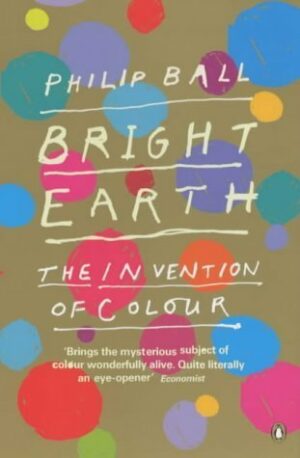